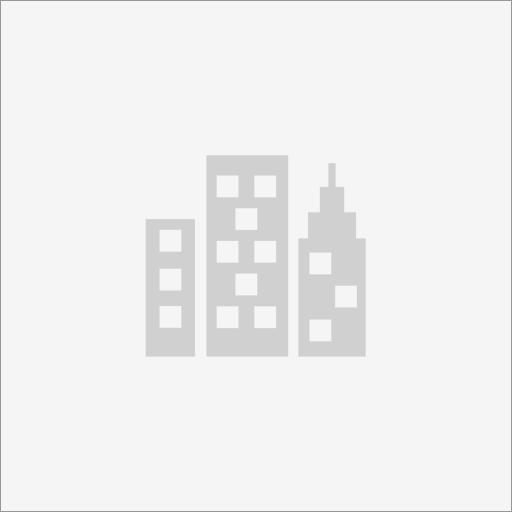
King’s College London
nearmejobs.eu
Cell migration is fundamental for the existence of multicellular animals, including humans, as certain cells migrate throughout the embryo to form specific tissues. It is also crucial for human health throughout life. To allow wounds to heal, fibroblasts must migrate to the wound. Similarly, cells of the immune system (neutrophils) migrate to wounds to clear them from pathogenic bacteria. To efficiently reach the wound, fibroblasts and neutrophils need to move directionally. Deregulation allows cancer cells to leave the primary tumour and to metastasise. Most cancer patients die after metastasis has taken place as metastases are hard to treat. For metastasis to occur, cancer cells need to migrate directionally to blood vessels to intravasate. Cancer cells, fibroblasts, and neutrophils can follow gradients of diffusible (chemotaxis) and/or immobilised cues (haptotaxis) to stay on their path for some time (cell persistence) to find their targets. We have a very limited understanding in general of how cells can control their direction and persistence.
Thus, one of the big questions in cancer research is how persistence is controlled during directed migration in non-transformed cells and how this regulation is altered in cancer cells. This information is crucial for development of specific drugs inhibiting metastasis in the future.
The polymerisation of the actin cytoskeleton network behind the plasma membrane in an area at the front of a cell, the lamellipodium, provides the pushing force for membrane protrusion and hence cell migration. We have already identified two key lamellipodial cytoskeletal regulators, Lamellipodin (Lpd) and Nance-Horan Syndrome-like 1 (NHSL1), which control random cell migration speed and persistence. We also found that Lpd promotes cancer invasion and metastasis (Law et al., Nature Communications, 2021; Carmona et al., Oncogene, 2016; Law et al., Journal of Cell Biology, 2013).
We hypothesise that cytoskeletal circuits control membrane protrusion and cell migration persistence and the directed migration of cells. Critically, motile cells are not controlled by linear pathways, but complex circuits.However, many current models of cell migration simulate only simplified cytoskeletal feedback loops because we do not know their exact molecular details.
Mathematical modelling of cell migration: Over the last years, we developed mathematical methods for modelling cell migration1–4. We based these on lamellipodium centered Turing-related positive and negative feedback circuits utilising reaction–diffusion equations similar to previous work5–8. We extended previous work by allowing for membrane bending rigidity alongside membrane tension and developed efficient and accurate solvers based on the evolving surface finite-element method1. We also developed parameter identification algorithms9,10 in which parameters in the model were estimated from experimental imaging data and for which the robust and efficient numerical method we designed was central.
In this interdisciplinary project, which will start in October 2024, you will develop a mathematical model of these crucial cytoskeletal circuits which regulate polarised lamellipodia protrusion and persistent cell migration: You will use an established mathematical framework for the modelling of cell motility and a numerical method for the simulation of this model in 2D. You will write reaction–diffusion partial differential equations for known and novel molecular interactions of cytoskeletal regulators of membrane protrusion dynamics at the circumference of cells using parameters that our interdisciplinary team obtained from their measurements to refine them coupled with an evolution law for the cell membrane. You will implement a numerical method, based on evolving triangulated surfaces, that consists of an evolving surface finite-element method for the approximation of the surface partial differential equation and a parametrized finite-element method for the approximation of the surface evolution law.
You will also remove interactions one by one in the model to predict key aspects of the circuits.
If you are interested, you can be also trained in the cell biological and microscopy techniques to contribute to the cell biology experiments of this project and get a full interdisciplinary training.
Taken together, your PhD work will unravel the differences in control mechanism of normal and cancer cell migration. This information will be crucial to develop specific drugs inhibiting metastasis in the future.
You will join two friendly and interactive labs and be co-supervised by Chandrasekhar Venkataraman, University of Sussex, Numerical Analysis and Scientific Computing Group (https://www.sussex.ac.uk/nasc/about) and Matthias Krause, Cellular Biophysics Section of the Randall Centre at King’s College London: 11 laboratories with shared interest in the regulation of the cytoskeleton in cell division, adhesion, migration, and intracellular trafficking with joint meetings. Furthermore, MKs lab is part of the London wide London Cell Motility Club which MK is organising.
To help us track our recruitment effort, please indicate in your email – cover/motivation letter where (nearmejobs.eu) you saw this posting.